views
Traditional cryptography, while historically essential, now faces evolving threats that necessitate the development and deployment of more sophisticated secur
ity paradigms. This demand has spurred the advancement of advanced cryptography, a field encompassing a range of innovative techniques designed to overcome the inherent limitations of conventional methods and provide enhanced functionality and security within increasingly complex digital environments.
Advanced cryptography transcends the basic principles of encryption and decryption, incorporating sophisticated techniques that enable operations on encrypted data, verification of data integrity without revealing its content, and secure multi-party computation without disclosing individual inputs. The fundamental principle is underpinning the minimization of data exposure in its plaintext form, thereby significantly reducing the attack surface and mitigating the risks associated with data breaches and unauthorized access.
The Inherent Constraints: Limitations of Traditional Cryptography
A thorough appreciation for the significance of advanced cryptography necessitates an understanding of the inherent limitations of its predecessor. Traditional cryptography primarily addresses confidentiality, achieved through encryption algorithms, and integrity, ensured through hashing and digital signatures. However, these techniques often require data decryption prior to processing or analysis, creating a critical window of vulnerability where sensitive information is exposed to potential threats.
Furthermore, traditional cryptographic methods are often designed for dyadic communication models. Consider a joint market research initiative involving several companies pooling their customer data; each entity is hesitant to reveal raw data yet seeks to derive collective insights. Traditional point-to-point cryptography struggles to effectively address such complex multi-party scenarios.
The emergence of disruptive technologies like cloud computing and the Internet of Things (IoT) further underscores the limitations of traditional cryptography. Cloud environments often involve data storage and processing on third-party managed servers, raising legitimate concerns regarding data privacy and security due to diminished user control. Similarly, the IoT ecosystem, characterized by a vast array of resource-constrained devices collecting and exchanging data, presents significant challenges for traditional cryptographic algorithms designed for more computationally powerful platforms.
Perhaps the most pressing challenge to traditional cryptography arises from the rapid advancements in quantum computing. Quantum computers possess the theoretical capability to execute certain computations exponentially faster than classical counterparts, thereby posing a significant threat to widely used public-key cryptographic algorithms, including RSA and Elliptic Curve Cryptography (ECC). This imminent threat has spurred intensive global research and development efforts in the domain of post-quantum cryptography, a critical sub-discipline within the broader framework of advanced cryptography.
Core Methodologies: Key Techniques in Advanced Cryptography
The field of advanced cryptography encompasses a diverse range of techniques, each offering unique strengths and applications in addressing the limitations of traditional cryptography across various dimensions of data privacy, security, and computation.
Homomorphic Encryption (HE): This revolutionary technique enables computations to be performed on encrypted data without requiring decryption. The encrypted result, when decrypted, yields the same output as if the operations had been performed on the original plaintext. While Fully Homomorphic Encryption (FHE), which allows arbitrary computations, remains computationally intensive, ongoing research is steadily improving its practicality and efficiency.
Post-Quantum Cryptography (PQC): The potential for quantum computers to compromise current cryptographic infrastructure necessitates the development of algorithms resistant to both classical and quantum attacks. Post-quantum cryptography focuses on creating and standardizing such algorithms based on mathematical problems believed to be intractable for quantum computers. Prominent candidate algorithms, currently under rigorous evaluation by organizations like the National Institute of Standards and Technology (NIST), include lattice-based, code-based, multivariate, hash-based, and isogeny-based cryptosystems, ensuring the long-term advanced security of digital systems in the anticipated post-quantum era.
Multi-Party Computation (MPC): In numerous real-world scenarios, multiple parties need to collaboratively compute a function over their private inputs while keeping those inputs secret. MPC provides cryptographic protocols enabling such joint computation, where each party contributes their data, and the result is revealed without any party learning the others' private information beyond what can be inferred from the output.
Elliptic Curve Cryptography (ECC): While not always strictly categorized as "advanced" in the same vein as HE or PQC, ECC represents a significant advancement over earlier public-key methods, offering comparable security levels with substantially shorter key lengths. This efficiency makes ECC particularly well-suited for resource-constrained devices prevalent in mobile computing, embedded systems, and the Internet of Things (IoT).
Emerging Paradigms: Further Techniques in Advanced Cryptography
Beyond these core methodologies, several other notable techniques contribute to the evolving landscape of advanced security:
- Private Information Retrieval (PIR): Enables a user to retrieve specific information from a database without revealing which information is being accessed.
- Zero-Knowledge Proofs (ZKP): Allow one party to prove the knowledge of a specific piece of information to another party without revealing the information itself.
- Private Set Intersection (PSI): Enables multiple parties to compute the intersection of their datasets without revealing any information beyond the common elements.
- Attribute-Based Encryption (ABE): Facilitates fine-grained access control to encrypted data based on the attributes of the user.
Charting the Course: The Future of Data Security
Advanced cryptography is not a static field but a dynamic and rapidly evolving domain with profound implications for the future of data security. From securing financial transactions and protecting sensitive personal data to enabling secure multi-party collaboration and mitigating the impending quantum computing threat, advanced cryptography is instrumental in forging a more secure, privacy-preserving, and resilient digital future.
Empower your professional trajectory in the dynamic landscape of information technology. Win in Life Academy offers a comprehensive suite of courses tailored for IT professionals seeking to cultivate expertise in cutting-edge technologies. Visit https://wininlifeacademy.com/ to explore our diverse curriculum and discover how we can facilitate your professional growth and mastery of critical IT domains.
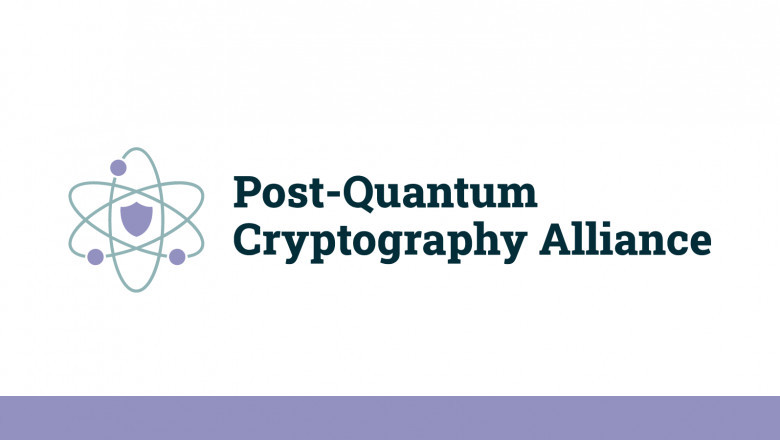

Comments
0 comment